- StudySolver
- /History of Treg Discovery
History of Treg Discovery
1 INTRODUCTION
1.1 History of Treg discovery
The adaptive immune system of higher invertebrates has facilitated a more efficient and specific defence against pathogens than that afforded by innate immunity alone. The hallmark of adaptive immunity is the generation of antigen receptors capable of developing lymphocyte clones through somatic cell gene rearrangement. This gives rise to essentially unlimited antigen-specific clones, creating a robust counter defence to rapid reproduction and high mutation rates of pathogens. However, the random and high diversity of this system also increases the risk of autoimmunity. This risk is further exacerbated by the requirement of the T cell receptor (TCR) recognition of major histocompatibility complex (MHC), which have loaded self-peptide. To overcome this issue, a ‘regulatory’ system must exist to delete autoreactive T and B cell clones both during development and in the periphery (reviewed in (Fontenot and Rudensky, 2005).
The first indication of ‘autoimmunity’ was proposed over a hundred years ago by Paul Ehrlich, suggesting a regulatory system must exist to overcome the issue of autoreactivity (Ehrlich, 1910). In 1969, the observation that mice which had undergone a thymectomy early in life went on to develop organ-specific pathologies was T cell-dependent autoimmunity characterised (Nishizuka and Sakakura, 1969). Additionally, this autoimmunity was shown to be preventable through adoptive transfer of cells derived from adult spleens. Conversely, T cells from autoimmune mice could transfer the disease to syngeneic T cell deficient mice (Penhale et al., 1973). These observations of autoimmunity were closely followed by a seminal discovery whereby a subset of CD4+ T cells, different to the ‘T helper’ phenotype, dampened an immune response during antigen challenge and were termed ‘suppressor’ T cells (Gershon and Kondo, 1971). These discoveries led to intense research across many immunological fields but a lack of easily distinguishable cellular markers, lack of clinical evidence in immunological pathology, and ambiguity in the molecular method of suppression, led to an abrupt decline during the mid-1980s in studying these ‘suppressor’ cells (Sakaguchi, 2004).
The field of suppression gained renewed interest with emerging molecular characterisations of the immunosuppressive cytokine interleukin 10 (IL-10), which gave evidence to asuppressive mechanism (Asseman et al., 1999a; Howard et al., 1992). The next major challenge was separating out T cells that all expressed CD4. Attempts to answer this challenge led to initial discoveries of CD4+ CD5hi and CD4+ CD45RBlo+ which when fractionated were shown to mediate autoimmunity (Reviewed in (Shevach, 2000). In 1995, CD4+ cells expressing the interleukin 2 receptor-α (IL-2Rα) chain, CD25, were identified as being highly ‘enriched’ in suppressor activity. CD4+ CD25+ cells were confined to CD5hi CD45RBlo expression and were found to constitute 5-10% of CD4+ peripheral T cells (Sakaguchi et al., 1995). This population was also the most suppressive and led to them being termed ‘regulatory’ T cells (Tregs).
Although CD25+ became the mainstay for defining non-activated Tregs in mice and humans, complete discrimination became difficult between Tregs and recently activated non-regulatory T cells. Furthermore, recently defined suppressive markers such as cytotoxic T lymphocyte-associated antigen 4 (CTLA-4), program death 1 (PD-1), and glucocorticoid-inducible tumour necrosis factor receptor (GITR) were also transiently upregulated on non-regulatory T cells (reviewed in (Tanaka and Sakaguchi, 2017). This suggests that these markers do not define the role of Tregs, but also may not be required for Treg function. This led to two theories of Treg functionality; first that a distinct population of Tregs existed under a genetic lineage-restricted program (Figure 1A), or Tregs represented a ‘plastic’ phenotype whereby environmental and cellular cues led to activated T cells differentiating into Tregs (Figure 1B) (Fontenot and Rudensky, 2005). The first model suggested for a mechanism of specifying Treg lineage, while the second model implied that T cell mediated immunosuppression was a dynamic balance between cytokine and receptor profiles (Stockinger et al., 2001).
Figure 1: Proposed models of dominant tolerance by Tregs. (A) A subset of Tregs are lineage restricted through fixed expression of FoxP3. Activation of effector T cells (FoxP3- CD4/8+) produced IL-2 which leads to expansion of the Treg population. Tregs then modulate the activated T cells. (B) Tregs are generated both thymically and de novo from effector non-regulatory T cells. Induction of FoxP3 in non-regulatory T cells acts as a negative transcriptional regulator of activated T cells, rather than as a specification marker.
A fatal autoimmune disorder ‘immune dysregulation, polyendocrinopathy, enteropathy, X-linked’ (IPEX) led to discovering mutations in the gene encoding FOXP3 (Bennett et al., 2001; Chatila et al., 2000). An analogous disease in mice, scurfy, also supported the model of a dedicated lineage of Tregs (Brunkow et al., 2001). FOXP3 is part of the large family of transcription factors defined by their winged helix-forkhead DNA-binding domain (forkhead box; FOX). FOXP3 is highly conserved between humans, mice and rats, suggesting its role in immunity is pivotal (Kim and Leonard, 2007; Mantel et al., 2006). Experiments using mouse bone marrow-derived stem cells deficient in the FoxP3 gene showed the stem cells could not give rise to CD25+ CD4+ Tregs, suggesting FoxP3 was essential in Treg development. Consistent with this observation, overexpression of FoxP3 gave rise to higher frequencies of CD4+ CD25+ Tregs, as well as higher suppressive capabilities of CD25- CD4+ and CD8+ T cells. This also provided evidence of the ‘plastic’ state of Tregs. It is now accepted that a distinct population of Tregs develop from the thymus (tTreg) where FoxP3 is essential in their development, however, Tregs can also be induced in the periphery (pTreg) which helps further control T cell immune responses and autoreactivity that evaded clonal deletion during development. Reviewed in (Li and Rudensky, 2016).
1.2 Thymic-derived Treg cell development
During both neonatal and adult life, hematopoietic stem cells migrate from the bone marrow into the thymus where they undergo positive and negative selection during TCR rearrangement. Within the thymus, the immature T cells, known as thymocytes, reside within the cortex of the thymus (Apostolou et al., 2002). Immature CD4+CD8+ double-positive (DP) T cells interact with cortical thymic epithelial cells (cTECs) and bone marrow-derived antigen presenting cells (APCs) which present self-antigens to the thymocytes (Jordan et al., 2001). Thymocytes with high affinity for self-antigen classically undergo clonal deletion. This is dependent on the apoptotic protein Bcl-2-like protein 11 (BIM). Studies on BIM-deficient mice revealed that approximately 56% of double positive(DP) and 20-60% of single positive (SP) T cells are clonally deleted in the cortex and medulla, respectively (Daley et al., 2013). Thymocytes with low-affinity TCR are positively selected for and differentiate into CD4+ or CD8+ SP cells which migrate from the cortex to the medulla. SP thymocytes continue to sample antigens presented by medullary TECs (mTECs) and DCs. The transcription factor autoimmune regulator (AIRE) is expressed by mTECs which promotes tissue-specific peripheral antigen generation for the thymocytes to sample (Aschenbrenner et al., 2007). High stimulation by the mTECs can lead to SP thymocyte clonal deletion, but paradoxically, strong TCR signals can induce CD4 SP cells to differentiate into Tregs (Tanaka et al., 2010). Thymic-derived Treg development occurs exclusively in the medulla but the exact reasons why SP Tregs then migrate away from the thymus and enter the periphery remains unclear.
1.3 Peripheral-derived Treg cell development
The origin of peripheral Tregs (pTreg) is less clear than that of tTreg generation. This is partly due to the challenges associated with distinguishing pTregs from tTregs in a mixed population. The transcription factor Helios has been suggested to be highly expressed in tTregs, allowing for discrimination from FOXP3+ Helios- pTregs (Thornton et al., 2010). However, it remains unclear if it is a genuine marker for tTregs as Helios expression also increases upon T cell activation in non-regulatory T cells (Szurek et al., 2015). Additionally, there is contradicting evidence using TCR sequencing to distinguish Treg populations. Some studies looking at Treg function in the gut pointed toward a thymic origin, while other studies suggest the majority of Tregs within the gut are generated de novo (Cebula et al., 2013; Nishio et al., 2015; Tanoue et al., 2016).
Despite the challenges in finding the origin of pTregs, their existence and the molecular mechanisms underlying their development is well characterised. Peripheral induction of Tregs can lead to phenotypes that are indistinguishable from tTregs (Chen et al., 2003a). However, the mechanisms of induction do differ, specifically in the affinity and temporality of TCR stimulation. Both in vitro and in vivo studies have shown that transforming growth factor beta (TGF-β) and IL-2 causes pTreg generation (Chen et al., 2003a). pTregs are thought to be the main counter-balance to foreign microbes and food within the gut and at mucosal sites. Knockdowns of pTregs leads to increased inflammation at gut and mucosal sites, while tTreg generation was not impacted(Tanoue et al., 2016).
1.4 Molecular control of Treg development
Expression of FOXP3 is a distinctive feature of Tregs. Ectopic expression of FOXP3 alone can lead to suppressive phenotype in conventional T cells (Tconv) (Hori et al., 2003). Due to this, stringent regulation exists for homeostasis of suppression-mediated immune responses.
Comparative genomic approaches on human, rat and mouse genomes led to initial discoveries of three conserved non-coding sequences (CNS1, CNS2 and CNS3) on the FOXP3 locus; two enhancers and a promoter (Kim and Leonard, 2007; Zorn et al., 2006). A 4th enhancer was discovered later (CNS0) (Zheng et al., 2010). Intensive studies were done on the function of each CNS, notably by Rudensky et al. which systematically deleted each CNS to determine function. CNS1 was found to be largely involved in pTreg development, but dispensable for tTreg development. Deletion of CNS1 leads to a large reduction in gut-associated Tregs, in keeping. Conversely, deletion of CNS3 led to a marked reduction in tTregs. CNS3 is also suggested to be essential for pTreg development. CNS2 is found to be mainly involved in Treg stability, via demethylation, whereby CpG islands are highly demethylated only in functional Tregs. This has allowed CNS2 demethylation to become a mainstay of stable Treg phenotype. Deletion of CNS2 does not affect tTreg or pTreg development, but rather disrupts stability during proliferation. CNS0 is found to be a superenhancers and required for tTreg development (reviewed in (Lee and Lee, 2018).
Multiple transcription factors bind to the FOXP3 gene. These include, but not restricted to; c-Rel, NFAT, Foxo, Smad3, and Stat5 (Li et al., 2014; Long et al., 2009; Ouyang et al., 2010; Ruan et al., 2009). Most of these transcription factors require TCR activation. Stat5 induction occurs via IL-2 signalling. Il-2 signalling and Stat5 binding to CNS2 protect Treg identity from other intracellular signals, allowing heritable stability of FOXP3 (Yao et al., 2007). Importantly, transcription factors such as Nr4a2 and Nr4a3 are induced dependant on the strength of the TCR activation (Sekiya et al., 2013). This is one mechanism by which TCR strength directs Treg phenotype.
1.5 Epigenetic regulation of Tregs
CNS2 demethylation is a definitive marker of Treg cell lineage, exemplified by many FOXP3-inducing transcription factors not been able to bind to CNS2 without demethylation (Polansky et al., 2010). Interestingly, FOXP3 can be transiently induced without requiring CNS2 demethylation via histone modification. In vitro stimulation via TGF-β led to prominent trimethylation of H3K4 on the CNS1 region, but not demethylation on CNS2 (Polansky et al., 2010). However, the FOXP3 expression was found to be transient, and subsequently fell in the absence of exogenous TGF-β. H3K4 trimethylation is strongly correlated to FOXP3 expression and is only visible on the FOXP3 locus of fully differentiated Tregs (Floess et al., 2007). Other forms of histone modification exist. CNS3 shows monomethylation of H3K4 regardless of FOXP3 expression, defined as a ‘poised’ enhancer marker. Interestingly, naïve CD4+ T cells with negative FOXP3 expression maintain this poised status, suggested to allow for enhanced peripheral development of Tregs, and underlines the importance of CNS3 in pTreg development (Feng et al., 2015).
1.6 Heterogeneity of FOXP3+ T cells
Emerging evidence points towards a more ‘plastic’ state of FOXP3 expression in humans. These populations are heterogeneous in phenotype and function. Studies have shown that in virto TCR stimulation results in low level FOXP3 expression, however, this low expression does not confer suppressive qualities (Sakaguchi, 2004). Attempts are now being made to decipher suppressive and non-suppressive FOXP3 CD4+ T cells. It has been proposed that three subpopulations of FOXP3+ CD4+ tells exist in peripheral human blood; FOXP3lo CD45RA+CD25lo, termed naïve or resting Tregs, FOXP3hi CD45RA- CD25hi activated or effector Tregs, and FOXP3lo CD45RA- CD25lo non-Tregs which do not confer suppressive activity (Cretney et al., 2011; Tanaka and Sakaguchi, 2017). Multiple studies now implicated these subpopulations of FOXP3+ cells in cancers and disease, addressing the need for further characterisation of the various stages of Treg activation through FOXP3.
1.7 Role of Tregs in cancer
While Tregs are indispensable for immunological self-tolerance, the suppressive nature of Tregs can hamper immune responses to tumours. A major obstacle for tumour immunity is eliciting a response to tumour antigens, which for the most part are normal self-antigens or quasi-self-antigens with genetic mutations. This is evidenced by the variable efficacy rates of cancer vaccines alone (reviewed in(Tanaka and Sakaguchi, 2017).
Several studies have implicated Tregs in neck, breast, lung, liver, pancreas, gastrointestinal and ovarian cancers. Notably, the decreased ratio of CD8+ T cells to FOXP3+ T cells correlates with poor prognosis, particularly in breast, gastric and ovarian cancers, although some cancers are an exception to this dogma (Nishikawa and Sakaguchi, 2010). The negative impact of Tregs on tumour ablation was demonstrated experimentally using cell-depleting anti-CD25 antibodies, either by in vivo antibody administration into mice, or in vitro depletion followed by cell transfer into histocompatible T cell deficient mice. Both resulted in eradication of a variety of syngeneic tumour models. Additionally, an increase of CD8+ infiltration was seen and upon re-challenge elicited a more rapid response compared to the initial tumour response, giving indication of tumour-specific memory (Onizuka et al., 1999; Shimizu et al., 1999).
Recent progress in cancer immunotherapies have either directly or inadvertently targeted Treg suppressive functions, resulting in effective cancer elimination. The most characterised and tested immunotherapy of this kind is anti-CTLA-4 blockade, which has resulted in two fully humanised monoclonal antibodies entering the clinic; Ipilimumab and Tremelimumab (Robert et al., 2011).
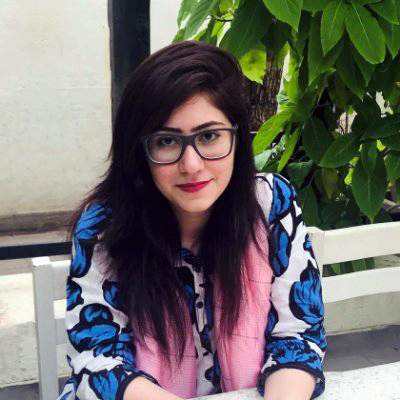
Freelance Writer
I’m a freelance writer with a bachelor’s degree in Journalism from Boston University. My work has been featured in publications like the L.A. Times, U.S. News and World Report, Farther Finance, Teen Vogue, Grammarly, The Startup, Mashable, Insider, Forbes, Writer (formerly Qordoba), MarketWatch, CNBC, and USA Today, among others.
Post a Comment
You must be logged in to post a comment.